Hydroxamic acids are a class of organic compounds having a general formula R−C−N−R’ bearing the functional group −C−N−, where R and R’ are typically organyl groups or hydrogen. They are amides wherein the nitrogen atom has a hydroxyl substituent. They are often used as metal chelators. They are more acidic than amides due to the presence of the hydroxyl group adjacent to the carbonyl, which allows for resonance stabilization of the conjugate base.
Common example of hydroxamic acid is aceto-N-methylhydroxamic acid (H3C−C(=O)−N(−OH)−CH3). Some uncommon examples of hydroxamic acids are formo-N-chlorohydroxamic acid (H−C(=O)−N(−OH)−Cl) and chloroformo-N-methylhydroxamic acid (Cl−C(=O)−N(−OH)−CH3).
Hydroxamic acids can be synthesized through several methods, one common approach involves the reaction of esters with hydroxylamine. Another method includes the treatment of carboxylic acids or their derivatives with hydroxylamine derivatives.
Structure of Hydroxamic Acid
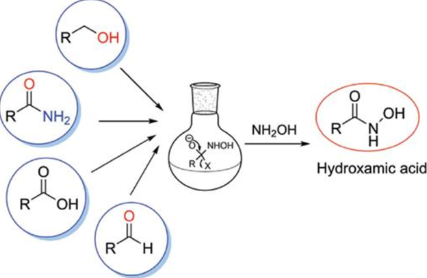
General Formula is R-CONHOH, where:
- R represents an organic substituent, which could be an alkyl, aryl, or any other organic group.
- C is a carbonyl carbon (C=O).
- NHOH is the hydroxamic acid group itself, consisting of a nitrogen atom bonded to a hydroxyl group (OH) and the carbonyl carbon.
Carbonyl Group (C=O): The carbon atom in this group is sp2 hybridized, forming a double bond with oxygen, which gives the molecule its planar structure around this area.
Hydroxylamine Group (-NHOH):
- Nitrogen (N): This atom is bonded to the carbonyl carbon, a hydrogen atom, and the hydroxyl group. The nitrogen typically has a trigonal pyramidal geometry if we consider its lone pair of electrons, although the presence of the carbonyl group can influence this due to resonance.
- Hydroxyl (OH): The oxygen here is bonded to both the nitrogen and a hydrogen atom. This group can participate in hydrogen bonding, both as a donor and acceptor.
Resonance and Stability
The hydroxamic acid group can exhibit resonance where the lone pair of electrons on the nitrogen can delocalize into the carbonyl group, leading to partial double bond character between the nitrogen and carbon. This resonance contributes to:
- Increased acidity: The resonance stabilization of the conjugate base makes hydroxamic acids more acidic than typical amides.
- Metal chelation: The ability to form stable chelates with metal ions, like iron, copper, etc., is enhanced by this electron delocalization, making the nitrogen and the carbonyl oxygen good coordination sites.
Physical Properties of Hydroxamic acids
Appearance: Typically, they are crystalline solids at room temperature. The color can vary from white to off-white or light yellow.
Melting Point: Varies widely with the nature of the R group but generally falls within the range of 70°C to 160°C for common hydroxamic acids.
Solubility: Generally soluble in polar organic solvents like DMSO, methanol, ethanol, and acetone. Solubility in water can be limited, but it increases with the presence of polar functional groups in the R moiety. Some are quite soluble due to the hydroxyl group which can form hydrogen bonds with water.
Acidity: They are weak acids. The pKa values are typically around 8 to 9, making them more acidic than simple amides due to the hydroxyl group adjacent to the carbonyl.
Stability: Hydroxamic acids can be sensitive to heat, light, and pH. They can degrade or hydrolyze back to their parent carboxylic acids and hydroxylamine under acidic or basic conditions.
Boiling Point: Decomposes before boiling in many cases, so boiling points are not commonly recorded or relevant due to thermal instability.
Density: Specific data can vary, but they are generally denser than water due to the presence of nitrogen and oxygen in a compact structure.
Odor: Most have no distinctive odor, but impurities or degradation products might introduce a slight odor.
Molecular Weight: Depends on the R group; for simple hydroxamic acids like acetohydroxamic acid, it’s relatively low, around 75.07 g/mol.
Reactivity: They can chelate metal ions, which is one of their notable chemical properties, useful in various applications like metal extraction or as enzyme inhibitors.
UV Absorption: They often show absorption in the UV spectrum due to the carbonyl group, with specifics depending on the R group’s nature.
Chemical Properties of Hydroxamic acids
Acidity: Hydroxamic acids are weak acids. Their acidity is due to the N-OH group, which can lose a proton, although they are less acidic than carboxylic acids.
Metal Chelation: One of their most significant properties is their ability to form complexes with metal ions, particularly with transition metals like iron, copper, and zinc. This property is utilized in analytical chemistry, medicine, and in the design of enzyme inhibitors.
Reactivity with Acids and Bases: Under acidic conditions, they can hydrolyze to form carboxylic acids and hydroxylamine. Under basic conditions, they can also undergo hydrolysis but might form salts due to their acidic nature.
Reduction and Oxidation: They can be reduced to amides using reducing agents like lithium aluminum hydride. Oxidation can lead to various products depending on the oxidizing agent; for instance, mild oxidation might lead to nitroso compounds.
Tautomerism: Hydroxamic acids exhibit tautomerism between the keto and enol forms, although the keto form (R-CONHOH) is generally more stable.
Nucleophilic Addition: The carbonyl group in hydroxamic acids can undergo nucleophilic addition reactions, similar to other carbonyl compounds.
Formation of Esters and Amides: They can react with alcohols to form hydroxamate esters and with amines to form N-substituted hydroxamic acids or undergo transamidation.
Thermal Decomposition: Upon heating, they might decompose, often leading to the formation of isocyanates among other products.
Complex Formation: Apart from simple chelation, they can form more complex structures like hydroxamates, which are used in siderophores by bacteria to capture iron.
Reactivity with Electrophiles: The nitrogen and oxygen atoms can both act as nucleophiles, reacting with electrophilic reagents. For example, they can be acylated or alkylated.
Biological Activity: Many hydroxamic acids exhibit biological activity, particularly as inhibitors of enzymes like histone deacetylases (HDACs), matrix metalloproteinases (MMPs), and ureases, due to their metal-binding capabilities.
Spectral Properties: They show characteristic bands in IR spectroscopy for O-H, N-H, and C=O stretches. In NMR, the chemical shifts of the protons adjacent to nitrogen and oxygen are distinctive.
Preparation of Hydroxamic Acid
From Carboxylic Acid Derivatives:
- Acid Chlorides: One of the most common methods involves reacting an acid chloride with hydroxylamine (NH₂OH). This reaction typically occurs under basic conditions to neutralize the hydrochloric acid produced:
RCOCl+NH2OH→RCONHOH+HCl - Esters: Hydroxamic acids can also be prepared from esters. Here, hydroxylamine reacts with an ester in the presence of a base:
RCOOR’+NH2OH→RCONHOH+R’OH - Anhydrides: Similar to acid chlorides, anhydrides can be used:
(RCO)2O+NH2OH→RCONHOH+RCOOH
Direct Amidation:
- From Carboxylic Acids: Direct conversion of carboxylic acids to hydroxamic acids can be achieved using coupling reagents like DCC (dicyclohexylcarbodiimide) or EDC (1-ethyl-3-(3-dimethylaminopropyl)carbodiimide), although this method might require activation of the carboxylic acid first:
RCOOH+NH2OH→Coupling Agent (RCONHOH)
From Nitriles:
- Hydrolysis of Nitriles: Nitriles can be converted to hydroxamic acids through partial hydrolysis or through the use of hydroxylamine:
RCN+NH2OH→RCONHOH
Oxidative Methods:
- Using Aldehydes: Aldehydes can be oxidized in the presence of hydroxylamine to form hydroxamic acids.
Biological Methods:
- Enzymatic Synthesis: Some enzymes can catalyze the formation of hydroxamic acids from various precursors, which is particularly interesting for green chemistry approaches.
Considerations
- Solvent Choice: The choice of solvent can greatly affect the reaction yield and purity. Often, reactions are carried out in solvents like methanol, ethanol, or THF.
- pH Control: Since many of these reactions involve or produce acids, controlling pH with bases like sodium hydroxide or triethylamine can be crucial.
- Purification: Hydroxamic acids might require purification through recrystallization or chromatography due to the formation of by-products.
- Safety: Hydroxylamine can be unstable or explosive in its pure form or when concentrated, so it’s often used as a salt (hydroxylamine hydrochloride) or in aqueous solution.
Uses of Hydroxamic Acid
Medical Applications:
- Chelation Therapy: Hydroxamic acids can bind to metal ions like iron and copper, which makes them useful in chelation therapy. For example, Deferasirox is a hydroxamic acid derivative used to treat iron overload in conditions like thalassemia.
- Anticancer Agents: Some hydroxamic acids, like Vorinostat (SAHA), are used as histone deacetylase (HDAC) inhibitors. These inhibitors can induce growth arrest, differentiation, or apoptosis in cancer cells, making them valuable in cancer treatment.
- Antibacterial and Antifungal: Their metal-chelating properties can disrupt microbial growth by depriving microorganisms of essential metal ions.
Industrial Uses:
- Flotation Collectors: In mining, hydroxamic acids are used as collectors in the flotation process to separate minerals. They selectively bind to certain metal ions, helping to extract valuable minerals from ores.
- Corrosion Inhibition: They can form protective films on metal surfaces, thus acting as corrosion inhibitors, particularly for steel in acidic environments.
Analytical Chemistry:
- Metal Extraction and Analysis: Hydroxamic acids are employed in solvent extraction processes to selectively extract and concentrate metals from solutions, which can be useful in both environmental analysis and in the recovery of metals from industrial waste.
Agricultural Applications:
- Pesticides: Some derivatives have been explored for their potential as pesticides due to their ability to interfere with metal-dependent enzymes in pests.
Research and Development:
- Enzyme Inhibitors: Their ability to inhibit various metalloenzymes makes them useful in biochemical research, where they help in understanding enzyme mechanisms or in developing new drugs.
- Synthesis: They are used in organic synthesis as reagents or intermediates due to their ability to participate in various chemical reactions, like the Lossen rearrangement.
Cosmetics:
- Though less common, some derivatives might find use in cosmetics for their potential chelating properties, which can help in stabilizing formulations or enhancing skin penetration of other ingredients.